COMPUTING
Hardware Implementation of Machine Learning
Deep learning has greatly advanced in recent years, but the hardware platform the machine learning algorithms run on has barely changed. A computer architecture that better supports machine learning than the traditional von-Neumann architecture is needed, and neuromorphic computing is one promising route. Neuromorphic computing emulates massive parallelism of human brain using electronic devices so that various contemporary and emerging machine learning tasks or those that deal with abundant data can be carried out more efficiently and faster. In this project, we are developing emerging non-volatile memory devices, including ReRAM (Resistive RAM), PCM (Phase Change Memory), fRAM (ferroelectric RAM), and MRAM (Magnetic RAM), and trying to emulate the behavior of biological synapses and neurons.
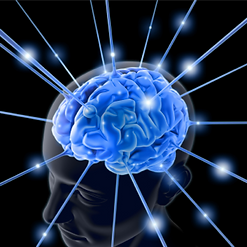
Emerging Semiconductor Materials and Devices
On 3/15/2024, the White House Office of Science and Technology Policy (OSTP) released a new strategy to strengthen the microelectronics and semiconductor research and development (R&D) innovation ecosystem in America. As called for in the bipartisan CHIPS for America Act, we proudly represent George Mason University as a leading research group for semiconductor and microelectronics. Being an active member of state-wide initiatives such as VAST (Virginia Alliance for Semiconductor Technology) and VMEC (Virginia MicroELectronics Consortium), Mason Nano Lab is conducting cutting-edge research on emerging semiconductor materials and devices. Current and future research efforts include materials and devices for steep-slope logic and high performance memory applications, and novel interconnect materials for chip-to-package connection.
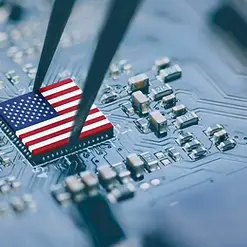
Quantum Materials and Devices
IBM, Google, and a few others have recently made a quantum computer based on superconducting circuits. However, the number of quantum bits (qubits) is still very few and there are still many challenges to overcome. Many research groups are thus looking into alternative quantum materials and devices baed on silicon quantum dot, ion traps, NV-center, or topological materials. Our group is conducting research on various low-dimensional nanomaterial systems (e.g., graphene, 2D materials) that are capable of spin-based quantum computation, including the Kitaev quantum spin liquid and heterostructures. The spin-spin and spin-orbit interactions are primarily investigated both experimentally and theoretically in terms of surface-induced decoherence and possibility for quantum hosting.

ENERGY
Low-dimensional Nanomaterials for Energy Harvesting
​
Unique functionalities of nanomaterials, such as piezoelectricity and thermoelectricity, can be harnessed for energy harvesting applications. We’re synthesizing and characterizing nanomaterials to help address one of the critical societal problems - producing electricity in an eco-friendly manner. For example, in our previous DoT-funded multi-institutional project, molybdenum disulfide was prepared by CVD at a large scale to be used as a renewable energy source for recharging the battery of electric or autonomous vehicles. Our group focuses on the development of practically-scalable energy harvesting devices that can be part of the useful system, in close collaborations with many other experts (materials scientists, circuit and IC designers, and systems engineers) in the field.
.jpg)
Multi-functional Oxides and Devices
Oxides have been typically used for insulating purposes, such as gate dielectrics in CMOS. However, our group is looking into the true potential of oxide thin films as the active, functional component of electronic devices. For example, our group recently published a pioneering work in Nanotechnology on the electrostrictive logic device based on the epitaxial oxide heterostructure. Once properly implemented, this novel device can tackle the theoretical limit of sub-threshold swing , thus contributing to the development of low-power electronic devices and ICs. A well-known memristive behavior of metal oxides is another example of how oxides can advance electronic devices of the future. Ferroelectric, piezoelectric, and ferromagnetic oxide thin films are in-house manufactured by using various PVD (physical vapor deposition) techniques.

High-performance Energy Storage Devices
Due to the intermittent nature of currently available energy harvesting technologies (e.g., photovoltaic, wind, etc.), one needs to save energy for future use. Li-ion battery is the incumbent, and researchers are trying to develop novel energy storage solutions for the future generation. Our group has the expertise in developing micro- and nanoscale, flexible, and all solid-state supercapacitor devices based on carbon nanostructures. Owing to the high surface-to-mass ratio of these nanomaterials, when they are used as electrodes, energy storage devices of both high energy density and high power density can be developed. We also develop an experimentally-benchmarked circuit model based on MATLAB/Cadence to simulate and predict the performance of these novel devices.

BIOMEDICAL
Microfluidic Sensors
​
We are leading a research project to develop microfluidic sensors using soft-lithography techniques. This technology finds many useful applications, including the biomedical sector. For example, we previously collaborated with UT Health Science Center at San Antonio (UTHSCSA) and US Army Institute of Surgical Research (USAISR) to fabricate high-spatial resolution tactile sensors for the development of haptic feedback devices for airway management. These sensors can be attached to a digital intubation device to ultimately reduce the number of survivable death casualties in the battlefield. This research project also has potential applications for robotic assisted surgery and space robotics.

Fiber-optic Microneedle Array
​
The fiber-optic microneedle array device is an array of hollow microneedles, capable of delivering vaccines or drugs to increase immunogenicity. The microneedles are made of bio-degradable materials in case it fractures inside the skin. Additionally, it can be designed to penetrate the stratum corneum but short enough not to pass the epidermis layer (where the nerve cells are located), thus reducing the chances of pain, infection or injury. This project employs the state-of-the-art microfabrication techniques to develop high aspect-ratio microneedles, in collaboration with scientists and engineers in other disciplines.
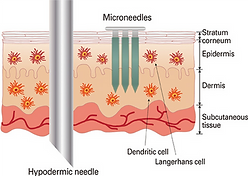